Light-matter interaction plays critical roles in experimental condensed matter physics and materials sciences, not only as experimental probes for revealing the underlying physics of low-dimensional quantum materials, but more importantly, as effective control knobs for manipulating the electronic structures and quantum states in the non-equilibrium state, with the fascinating opportunities to induce new physical phenomena beyond those in the equilibrium state.
In a paper entitled “Pseudospin-selective Floquet band engineering in black phosphorus” published inNature, researchers led by Prof. Shuyun Zhou from the Department of Physics at Tsinghua University reported the first experimental realization of light-induced transient manipulation of the electronic structure in a semiconductor – black phosphorus - via Floquet engineering. This work is the first experimental demonstration of Floquet band engineering in a semiconductor, which paves an important step toward light-induced emerging phenomena in quantum materials, such as topological phase transition via Floquet engineering.
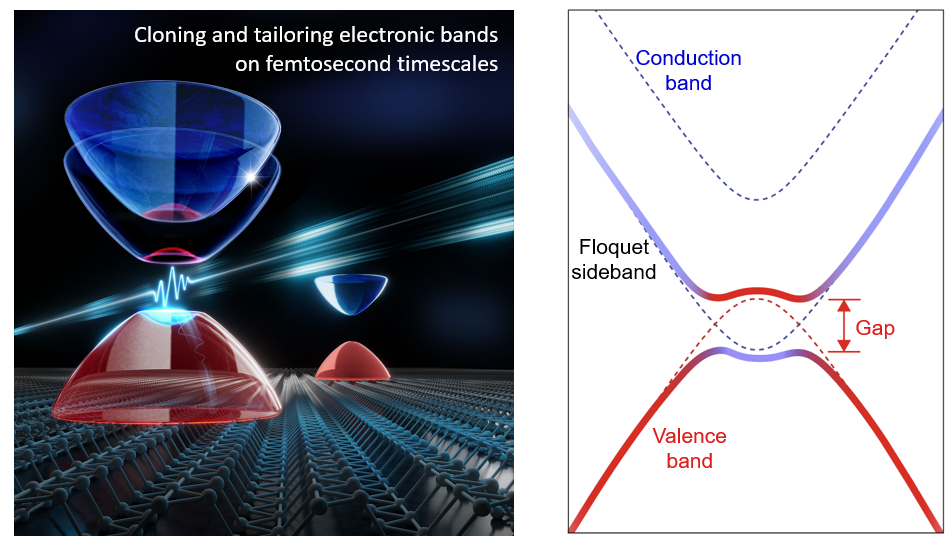
Figure 1: Schematics for Floquet band engineering in semiconductors
Low-dimensional quantum materials exhibit rich properties. So far, research about these materials has been focused mostly on their equilibrium properties, while the ultrafast dynamics in the non-equilibrium state are still in the developing stage. Capturing the transient electronic structure on ultrafast timescales (picosecond or even femtosecond, 10-12or 10-15seconds) and manipulating their nonequilibrium material properties is a fascinating field, which not only extends our knowledge about non-equilibrium physics, but also can lay important foundations for the development and application of future high-speed devices.
Time-periodic fields provide unprecedented opportunities for tailoring the quantum states of matter by Floquet engineering. In solids, the periodic arrangement of atoms leads to electronic structure, which is periodic in momentum. In analogy, time-periodic drive can lead to Floquet states, which are periodic in energy (Fig. 1). More importantly, the interaction between electrons in the material and time-periodic driving field can further lead to modifications of the electronic structure, symmetry, and topological properties, etc. Such Floquet engineering can result in light-induced emergent phenomena that are otherwise not possible in the equilibrium, for example, turning a topologically trivial material into a topological nontrivial material, realizing topological superconductivity far away from equilibrium, etc.
Floquet engineering has attracted extensive interest over the past few decades, and has been applied to condensed matter physics, cold atoms, and optical lattices ever since then. Nowadays, Floquet engineering has become an active and fast-developing research field in condensed matter physics and material science. However, in contrast to the rich theoretical predictions on intriguing light-induced topological phase changes, experimental progress along is extremely limited. Many fundamental questions still remain to be answered from experimental results. For example, can Floquet engineering be realized in conventional materials such as a semiconductor under realistic experimental conditions? Addressing questions like this will be a critical step toward the long-sought goal of light-induced emerging phenomena such as light-induced topological phase transition.
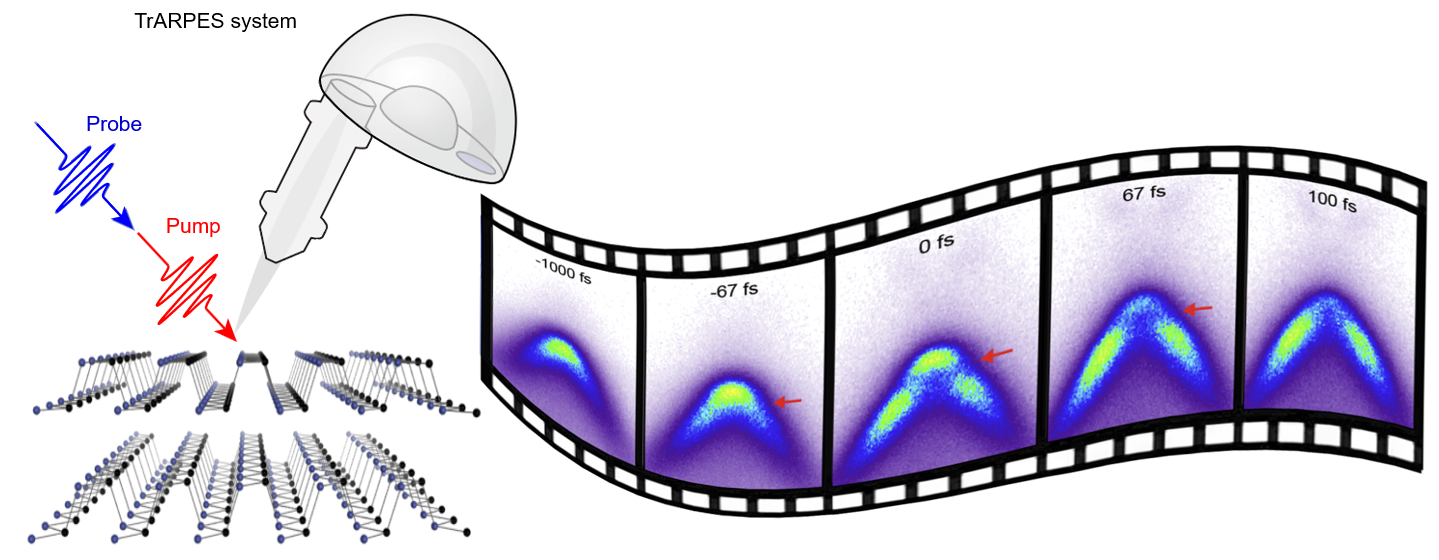
Figure 2: Experimental evidence of Floquet band engineering in black phosphorus by TrARPES. The arrows mark the light-induced gap opening in the transient electronic structure.
The experimental group led by Prof. Shuyun Zhou focuses on the electronic spectrum and ultrafast dynamics of two-dimensional materials and topological materials. Especially, they have been implementing instrumentations that are favorable for investigating light-induced emerging phenomena, and have been searching for experimental conditions realizing Floquet engineering in these materials. Because Floquet engineering requires low photon energy and a strong peak electric field, they have invested a lot of efforts to develop high-intensity mid-infrared pumping pulses. In addition to developing the state-of-the-art time- and angle-resolved photoemission spectroscopy (TrARPES) instrumentation, they have chosen a nearly-ideal semiconductor sample to start with – high-quality black phosphorus with a small band gap and high mobility. By fine tuning the photon energy, they found that upon near-resonance pumping, the band structure of black phosphorus evolves dramatically from a nearly-parabolic shape to a “Mexican hat” shape, indicating that light-induced hybridization gap opening (pointed by the red arrows in Fig. 2) in the transient electronic structure. The light-induced gap emerges simultaneously with the Floquet sidebands, and through a systematic investigation of the light-induced gap with delay time, pump fluence, and electron doping, they conclude that the light-induced gap is caused by Floquet band engineering, namely, light-induced manipulation of the transient electronic structure.
More interestingly, they found that the Floquet engineering in black phosphorus shows a strong pump polarization dependence: the Floquet engineering induced gap emerges only when the pump polarization is along the armchair direction of black phosphorus, suggesting that there are also optical selection rules for Floquet engineering. Combining these experimental results with theoretical analysis, they found that that the observed polarization selectivity originates from the coupling to the pseudospin degree of freedom (the two sublattices in black phosphorus can be viewed as a two-level system as spin). This work provides important insights for Floquet engineering of a semiconductor, and lays an important foundation for further exploring the transient manipulation of topological states, and correlated states such as magnetism and superconductivity.
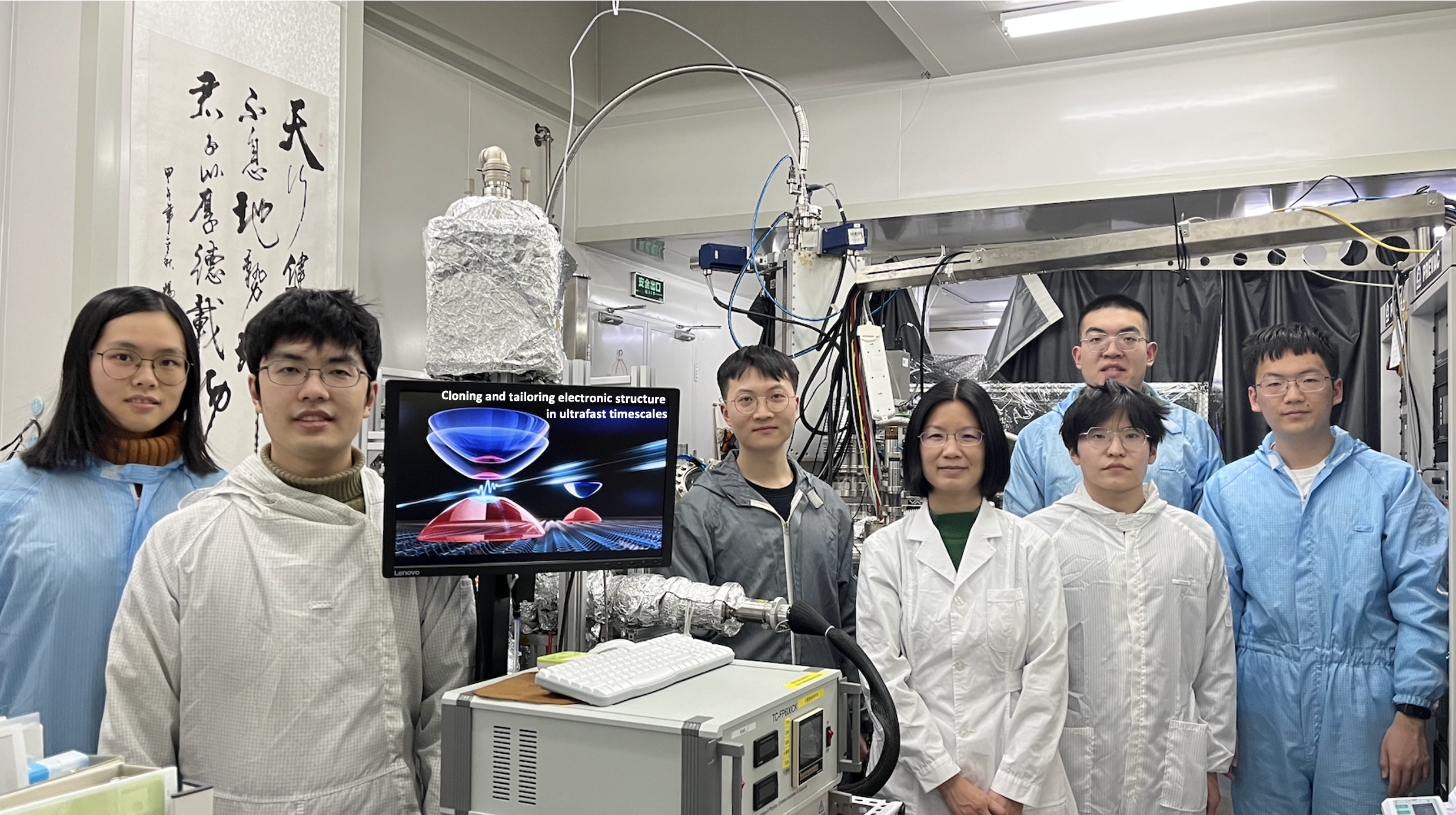
Figure 3: Members from Zhou group at Tsinghua University.
This work is a collaboration between Shuyun Zhou’s group at the Department of Physics, Tsinghua University, Pu Yu at Tsinghua university, and theoretical efforts from Wenhui Duan group at the Department of Physics, Tsinghua University, Peizhe Tang from Beihang University, and Sheng Meng group from Institute of Physics, Chinese Academy of Sciences.
This research was mainly supported by the National Key R&D Program of China (2021YFA1400100), the National Natural Science Foundation of China (11725418, 12234011, 11427903). Funding support from National Key R&D Program of China (2020YFA0308800, 2016YFA0301004, 2021YFA1400201), National Natural Science Foundation of China (51788104, 12025407) and Chinese Academy of Sciences (YSBR047) is also acknowledged.
Full-text article:https://www.nature.com/articles/s41586-022-05610-3
Shuyun Zhou group:http://info.phys.tsinghua.edu.cn/zhou/
Editor: Li Han